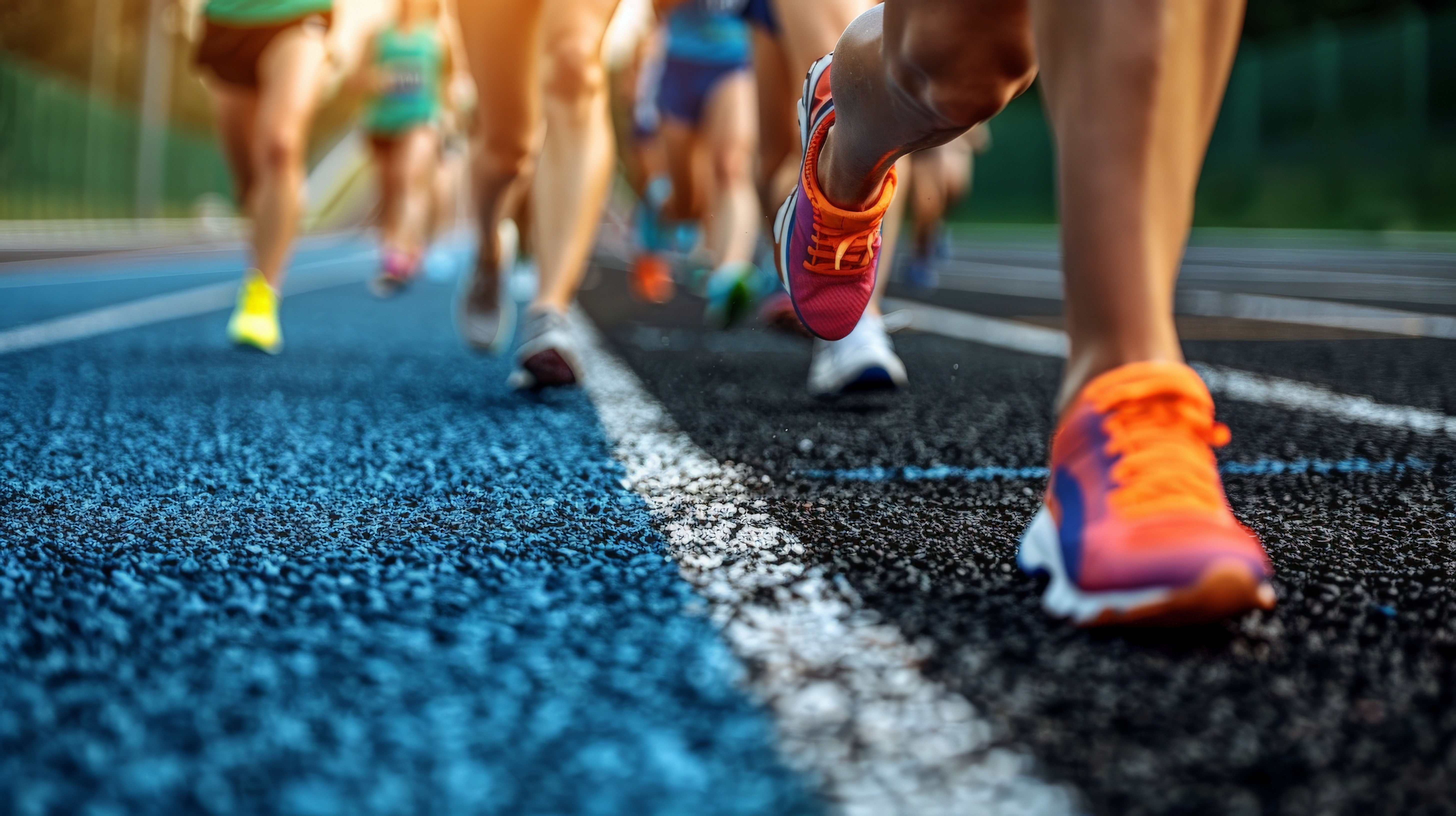
While bacteria have frequently made the medical news in recent years due to the urgent problem of antibiotic resistance, there is a growing movement trying to harness their capabilities for medical benefit, particularly in the field of oncology. We recently looked at how immunotherapy can be supplemented with faecal microbiota transplant (FMT) to improve its efficacy in melanoma treatment. But there are numerous other exciting approaches to the use of bacteria in cancer therapy.
A little-known fact is that the first attempt to treat cancer with bacteria occurred over a century ago, when William Coley trialled Streptococcus pyogenes (the causative agent of scarlet fever) in patients with inoperable tumours[i]. Although the trial was unsuccessful (and a number of patients died of their infections), some tumours were shown to regress, demonstrating the potential of microbial therapy in this context.
The first successful microbial therapy for cancer commenced in the 1970s, when Bacille de Calmette et Guérin (BCG), a strain of Mycobacterium bovis better known for its use as a vaccine against TB, began to be used for treatment of bladder cancer[ii]. Intravesical BCG therapy today remains the standard of care to prevent post-surgery relapse in non-invasive bladder cancer, although its mechanism of action remains unclear.
Bacterial treatments for cancer under development can be broadly categorised into two approaches: (i) exploiting the immune-stimulatory properties of bacteria to induce an immune response against tumours; and (ii) genetically engineering bacteria to deliver a therapeutic molecule to tumours. The two approaches can also be combined, by engineering bacteria to secrete immunomodulatory proteins (such as cytokines) to enhance their inherent immunostimulatory properties.
Bacterial immunostimulation
Many bacterial species and strains have been found to accumulate preferentially in solid tumours, compared to healthy tissue, presumably due to the nature of the tumour environment. In particular its immunosuppressive properties inhibit bacterial clearance by the immune system, and its hypoxic nature is particularly advantageous for proliferation of obligate and facultative anaerobes.
A key aim of modern cancer therapy is to stimulate patients’ immune systems to target and destroy their own tumours. The immunosuppressive nature of the tumour environment is a significant barrier to this. Bacteria, however, are highly immunostimulatory, as they produce a large number of pathogen-associated molecular patterns (PAMPs), which are recognised by pattern recognition receptors (PRRs) in innate immune cells such as monocytes and neutrophils. Activated innate immune cells recruit T cells to the target site, which are the primary immune cell type capable of attacking tumour tissue.
One promising approach to bacterial therapy for cancer is therefore to cause bacterial colonisation of a tumour, thereby stimulating an immune response in and against the tumour[iii].
The most advanced bacterial strain for this approach is Clostridium novyi-NT, an attenuated strain (NT = non-toxic) which has shown promise when injected directly into solid tumours. Like all Clostridia, C. novyi is a spore-forming obligate anaerobe. C. novyi spores administered to cancer patients preferentially germinate in the hypoxic environment of solid tumours, inducing an immune response in the tumour. In a mouse model, C. novyi therapy was found to be capable of inducing a complete response to solid tumours, and lasting anti-tumour immunity[iv]. A phase I clinical trial of C. novyi-NT for advanced solid tumours has been completed and showed promise, with over 40 % of patients showing a response to the therapy, though serious adverse events associated with C. novyi infection (sepsis, gas gangrene) were seen in over 10 % of patients[v].
Delivery of therapeutic proteins by bacteria
Bacteria have been engineered to deliver a wide range of therapeutics to solid tumours, including cytotoxins, immunomodulators, prodrug converting enzymes, siRNA and nanobodies.
Modification of bacteria to secrete immunostimulatory proteins has been found to substantially enhance the immune response they induce. A recent phase I study of metastatic gastrointestinal cancer treatment using avirulent Salmonella typhimurium modified to express human IL-2 demonstrated significant immune activation, though unfortunately no survival benefit[vi].
Other approaches which have shown promise in pre-clinical studies include the modification of E. coli to express the pore-forming cytotoxin cytolysin A for direct targeting of tumour cells, immune checkpoint inhibitors such as nanobodies against PD-1 or CTLA-4, or nanobodies against tumour-associated antigens such as CD47[vii].
There are many promising bacterial-based cancer therapies in development, utilising a wide array of strategies. Development of bacterial therapies is, however, associated with unique challenges not faced with traditional pharmaceuticals. For example, as is apparent from the C. novyi clinical trial described above, there is a risk of pathogenic infection, particularly in immunocompromised patients. Also, since bacteria are able to proliferate, bacterial therapies may not follow traditional pharmacokinetics, or display normal dose-response relationships. Dose calculation is therefore a challenge. Nonetheless, it is exciting to see the potential of this largely new therapeutic approach, and with many ongoing clinical trials in the space we are hopeful that we will soon see more bacterial therapies reach the clinic.
Ed handles patent work across the life sciences/biotech field, with a particular focus on antibody therapeutics, cell therapy and vaccines. Outside of the medical sphere he also has extensive experience in enzyme technology. Ed is very experienced in drafting patent applications and prosecuting them around the world, and also handles opposition and appeal work at the European Patent Office.
Email: edward.couchman@mewburn.com
Our IP specialists work at all stage of the IP life cycle and provide strategic advice about patent, trade mark and registered designs, as well as any IP-related disputes and legal and commercial requirements.
Our peopleWe have an easily-accessible office in central London, as well as a number of regional offices throughout the UK and an office in Munich, Germany. We’d love to hear from you, so please get in touch.
Get in touch