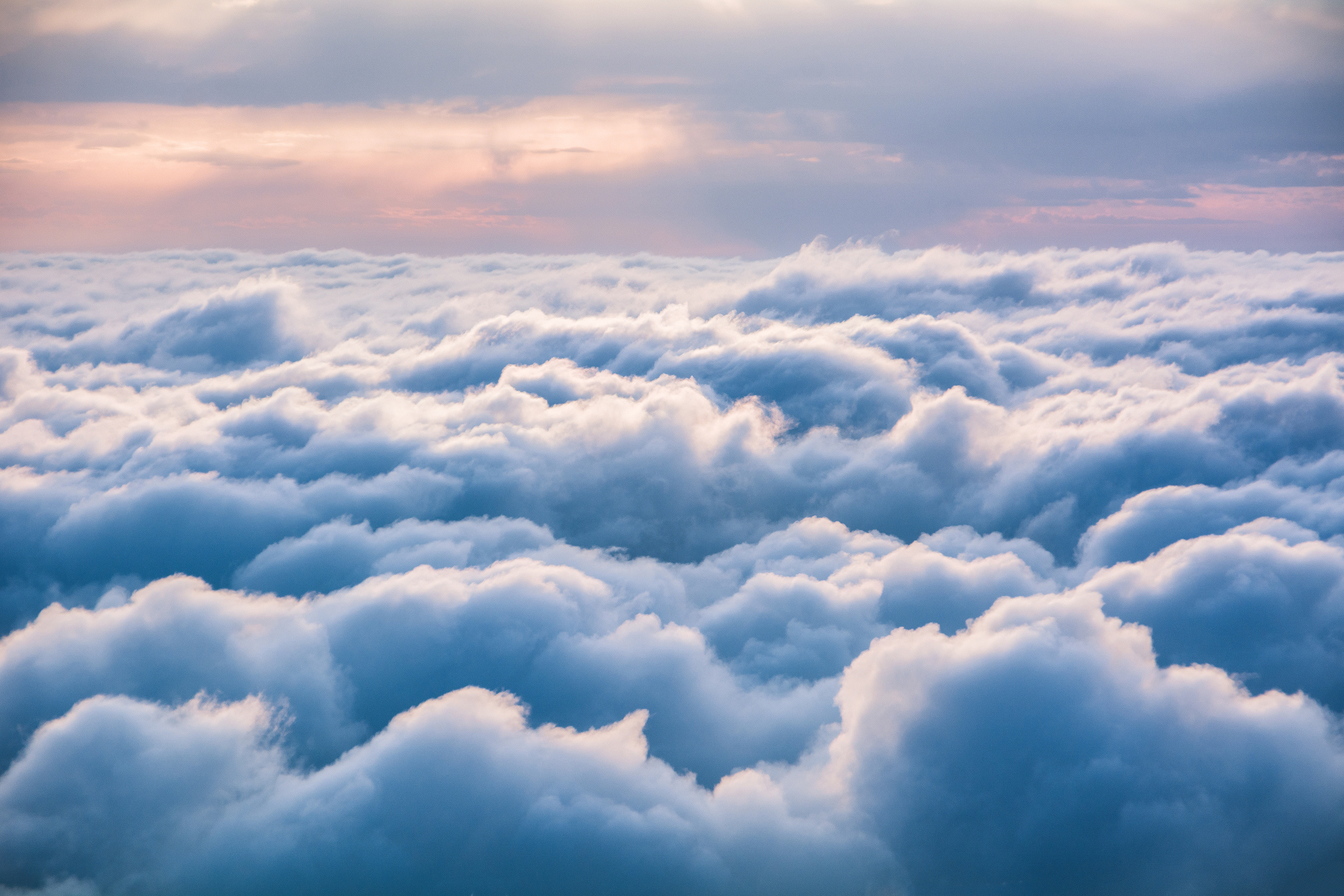
The human genome contains roughly 20,000 genes that encode proteins. In order for the cells and tissues of a human to function properly, the expression of these genes and the activity of the proteins that they encode must be kept under close control. The mechanisms that provide this control are complex and defects frequently result in the onset of diseases, such as cancer. Because these defects are often reversible, control mechanisms have attracted considerable interest as targets for the development of new drugs.
Research into the chemical modification of DNA and proteins are already well-established fields. A new emerging field of epitranscriptomics focusses on the chemical modification of RNA molecules in cells. Recent advances have shed light on the mechanisms that add, remove and interact with these modifications and the manipulation of these mechanisms is opening up promising new avenues for the development of new drugs. In this blog we explore these different mechanisms used for controlling proteins and DNA and take a look at the exciting new field of epitranscriptomics.
Post-translational mechanisms control the activity of proteins that are already present in a cell. Typically, these mechanisms involve introducing chemical modifications to the protein that alter its structure. For example, a chemical group, such as a phosphoryl, acetyl or ubiquitinyl group, may be covalently linked to the protein to alter its activity, for example, by switching it on or off. Interfering with these mechanisms, for example by inhibiting the enzymes that add or remove chemical groups, can alter the activity of a protein. This has numerous medical applications. For example, enzymes that attach phosphoryl groups to proteins (known as kinases) are the targets of at least 35 drugs that have been approved for the treatment of cancer1.
Mechanisms that regulate gene expression control the location and timing of the production of a protein. These mechanisms include the chemical modification of genomic DNA, which is transcribed within the cell nucleus into RNA in the first step of gene expression. Most of the modifications identified to date involve the addition of chemical groups, such as methyl, hydoxymethyl or formyl groups to the 5’-carbon of cytosine bases in the DNA2. Cytosine methylation in particular has been found to inhibit transcription and switch off the expression of genes. The inhibition of enzymes that methylate cytosine (known as DNA methyltransferases or DNMTs) switches on the expression of genes that were previously switched off. This can be useful in therapeutic settings and a number of drugs that target DNMTs, including azacytidine, are currently being investigated as cancer therapeutics.
Modification of DNA is not the only control mechanism for gene expression. Proteins concerned with the packaging of genomic DNA, including histones and other chromatin proteins, may also be reversibly chemically modified in order to regulate expression. Chemical modifications may include the addition of chemical groups, such as methyl, acetyl or ubiquitinyl groups, to any of a number of sites within the histone structure. The addition and removal of these groups winds or unwinds the coiling of the genomic DNA around the histones and either activates or inhibits transcription. A suite of enzymes, including histone acetyl transferases (HATs), histone deacetylases (HDACs) and histone methyltransferases3 (HMTs) are responsible for adding and removing chemical groups from chromatin proteins and many of these enzymes have been associated with diseases, such as cancer. This makes them attractive drug targets and at least ten different HDAC inhibitors have been approved or are clinical trials for the treatment of various types of cancer, including cutaneous T cell lymphoma4.
In addition to the modification of DNA and protein, it is becoming increasing clear that gene expression is also controlled by the modification of RNA. This exciting field is taking medical research by storm as there are potentially many more intervention points with RNA modifications than with DNA modifications.
Reversible chemical modification of bases within an mRNA molecule may alter the splicing of the mRNA molecule or its nuclear export, translation or degradation and play an important role in determining the extent to which the mRNA is translated into protein by ribosomes within the cell. The reversible modification of RNA is known as epitranscriptomics.
Over a hundred different chemical modifications exist within human RNA. These include modifications include the addition of chemical groups to bases within the RNA molecule or alterations in the ring structure of the base. Large families of RNA modifying enzymes are responsible for these modifications and each modification is added, removed or read by a different set of writing, erasing and reading enzymes. However, only a few of these RNA modifying enzymes have been well-characterised to date.
One of the best studied modified RNA bases is 6-methyladenosine (m6A). 6-methyladenosine is found in mRNA, pre-miRNA and long noncoding RNA, and regulates various aspects of mRNA processing, including splicing, export, stability and translation. 6-methyladenosine is generated by addition of a methyl group to the nitrogen-6 position of adenosine by the writer enzymes METTL3 and 14. The methyl group of m6A is removed by the eraser enzymes FTO and ALKBH5. A number of reader proteins including YTH domain-containing proteins 1 and 2 (YTHDC1 and YTHDC2) and YTH domain-containing family proteins 1, 2 and 3 (YTHDF1, YTHDF2 and YTHDF3) are known to bind to RNA binding sites containing m6A and these reader proteins are thought to mediate its activity. Although the presence of m6A is known to affect differentiation, its precise role is currently unclear and reduced levels of m6A have been shown to inhibit differentiation in some systems and promote differentiation in others.
Like protein and DNA modification, it is becoming increasingly apparent that RNA modification in disease condition. Cancer cells often show up-regulation of RNA writers, erasers and readers5 and m6A has recently been associated with certain cancers6, including acute myeloid leukaemia (AML) and glioblastoma. This association has made the writers, erasers and readers of m6A modification hot targets for therapeutic intervention. In particular, METTL3, which “writes” m6A modifications, has recently been found to be essential for the growth of acute myeloid leukaemia (AML) cells7, 8. A number of biotechnology companies, including Storm Therapeutics in the UK and Gotham Therapeutics and Accent Therapeutics in the US, are racing to develop drugs that target METTL3 as potential treatments for certain types of cancer.
There are still many unanswered questions about the mechanics of RNA modification and its role in disease. However, the emerging picture of epitranscriptomics as a fundamental mechanism for regulating gene expression suggests that RNA modifying enzymes will become a significant new class of drug targets for cancer and other diseases. At Mewburn Ellis, we have a long track record of building robust IP portfolios around new drugs and drug targets, and are excited at the opportunity to support the commercialisation of the innovations will arise as the field of epitranscriptomics develops.
1 Bhullar et al Mol Cancer 17 48
2 Kumar et al Front. Genet., 2018 https://doi.org/10.3389/fgene.2018.00640
3 Morera et al Clin Epigenetics. 2016; 8: 57
4 Suraweera et al Front Oncol. 2018; 8: 92
5 Lan et al Cancer Res 2019 79 7
6 Chem et al Mol Cancer 2019 18 103
7 Barbieri, I. et al. Nature 552, 126–131 (2017)
8 Weng, H. et al. Cell Stem Cell 22, 191–205.e9 (2018)
Nick is a Partner, Patent Attorney and Litigator at Mewburn Ellis. He works across the full range of patent activity in the life sciences sector, from pre-drafting advice and drafting of applications to worldwide portfolio management, prosecution and appeal. Nick is also experienced in defensive and offensive European oppositions and due diligence work.
Email: nick.sutcliffe@mewburn.com
Our IP specialists work at all stage of the IP life cycle and provide strategic advice about patent, trade mark and registered designs, as well as any IP-related disputes and legal and commercial requirements.
Our peopleWe have an easily-accessible office in central London, as well as a number of regional offices throughout the UK and an office in Munich, Germany. We’d love to hear from you, so please get in touch.
Get in touch