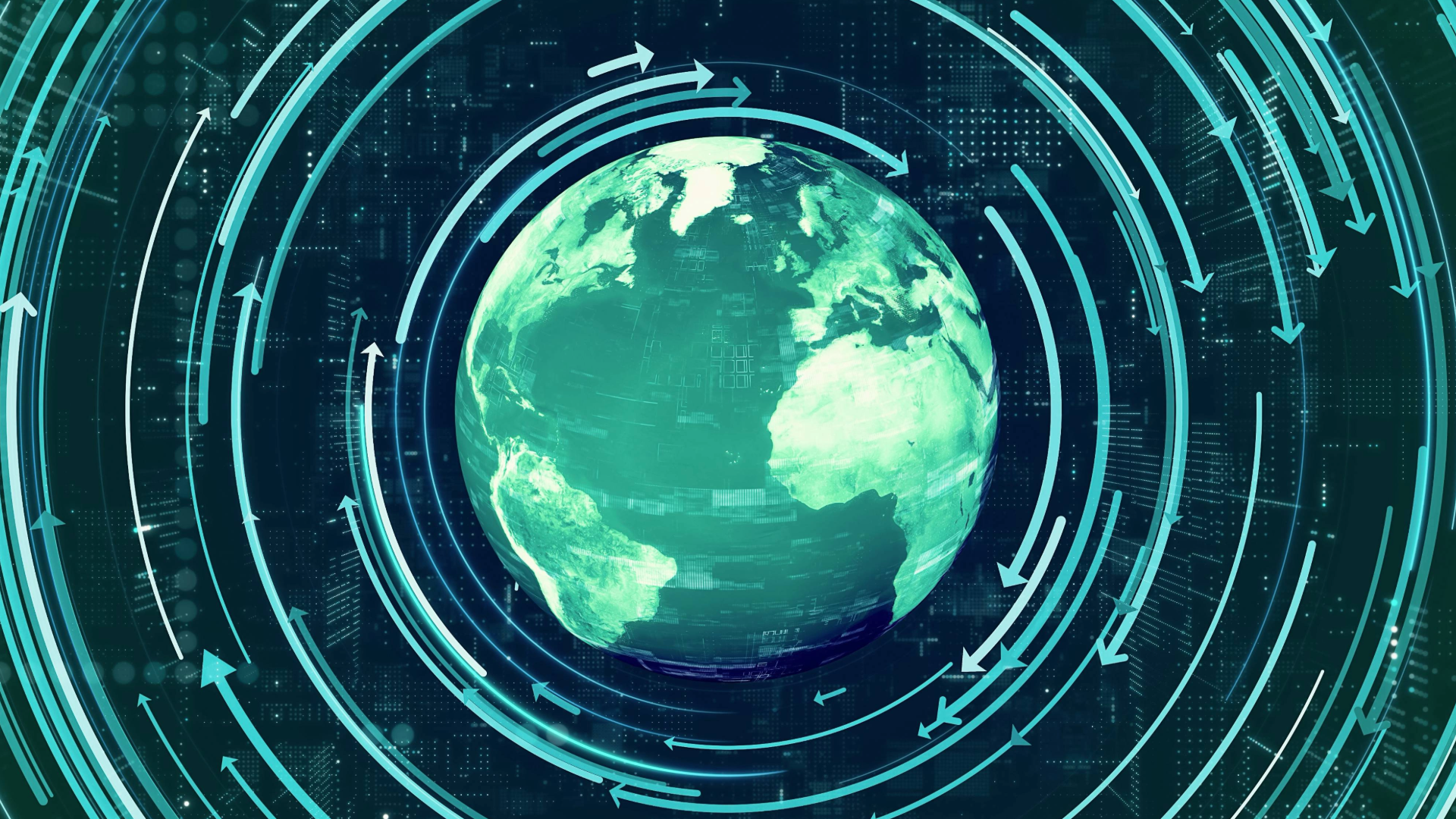
Methane, the principal component in natural gas, accounts for approximately a third of the planetary warming being experienced today1. Whilst it may have a shorter lifetime than CO2, methane is significantly more potent, having a warming impact around 80 times stronger per unit of mass over a 20 year period2.
Whilst methane has a variety of both anthropogenic and natural sources, human activity contributes significantly to methane production. Three major human-originating methane sources are:
Whilst it isn’t straightforward to capture methane produced by cows (although that isn’t stopping some innovators trying!), the fossil fuel industry still accounts for about 35% of anthropogenic methane emissions2. This is an industry ripe for easy methane capture, and to meet global targets changes must be implemented soon. A UNEP report suggests that, to reach the 1.5°C reduction set by the IPCC, the methane emissions from this sector must be 30-60% lower than the levels recorded in 2020 come the decade’s end1.
The question remains: once we capture all this methane, what can be done with it? Along with the increasing need to cut methane emissions, there’s an equally increasing demand for carbon-based nanomaterials. Innovators across the globe have been hard at work marrying the solutions to both of these problems.
Methane has a rather simple chemical formula, CH4, and so like many of today’s fuels it is made from carbon and hydrogen. Problematic when bonded together, these constituent parts can be invaluable once separated. Separation is no easy feat: breaking the four C-H bonds is an endothermic process requiring significant amounts of energy.
At the time of writing, there are two primary ways this can be done:
The front-runner in hydrogen gas production, SMR harnesses two stages of chemical reactions between natural gas (mainly methane) and steam to yield hydrogen and carbon dioxide3. This is done at high temperatures and usually in the presence of a catalyst. Whilst this is a relatively cheap and extremely effective way to produce hydrogen gas, a glaring problem remains: the CO2 by-product. In the long run trading methane for CO2 will not help with global temperature targets.
Whilst the production of one greenhouse gas (GHG) in the place of another is not ideal, it is not impossible to deal with. Johnson Matthey for example is able to capture up to 95% of carbon dioxide produced in SMR using their CLEANPACE™ technology. This form of carbon capture is fantastically efficient but, as a storage solution, we’re still missing the utility of all that extra carbon.
A relatively new player to the game, pyrolysis represents a promising avenue for hydrogen production with reduced carbon gas emissions. Instead of reacting the methane with steam, high temperatures are used to brute force the bonds apart, reducing methane to its constituent elements – hydrogen and solid carbon3. Pyrolysis therefore paves the way for GHG-free hydrogen production. The solid carbon produced is typically either in the form of graphite or a material called carbon black (a common additive from rubber to lithium-ion batteries). These are often seen as low-quality carbon materials and are commonly a mixing pot of randomised, disordered structures5.
Conventionally, pyrolysis is done in a simple heating chamber capable of pushing the methane to temperatures in the range of 900-1400°C. Such high temperatures can be reduced considerably with the use of a catalyst4, but this comes with the added cost and logistical challenge of separating the solid carbon from the catalyst afterwards.
More energy efficient still is the use of plasma as a heat source. Aptly named plasma pyrolysis, this technique takes advantage of the high-temperature arc produced between two high-voltage electrodes. When the arc is exposed to compressed air, a pressurised jet of plasma is produced. As plasma pyrolysis relies on electricity rather than combustion to heat methane, there is the potential for completely green methane cracking when leveraged with renewably sourced power.
The standard outputs of the plasma pyrolysis of methane are hydrogen and the low-quality carbon materials previously mentioned; however, there are experimental and commercial developments kickstarting the replacement of carbon black as a by-product with sought-after carbon-based nanomaterials.
The Global Hydrogen Production Technologies Center (HyPT) is an international partnership combining the research efforts of institutions from 6 different countries to transform the production of net zero hydrogen.
Professor Adam Boies and his research group in Cambridge have also been working to tackle this problem. The group specialises in the formation of carbon nanotubes from floating nanoparticle catalysts, the process dubbed floating catalyst chemical vapour deposition (FCCVD). This method is a scalable, gas-phase, single-step route for producing assembled carbon nanotube material products. It involves incorporating an iron and sulphur source in the methane cracking process, wherein the thermal decomposition of these additives yields nanoparticle catalysts - minute spherical surfaces for the CNTs to grow upon. These nanotubes clump together into a tapestry of carbon nanotube aerogel, which can then be continuously drawn out of the reactor and spooled6. CNTs at the nanoscale boast superior properties to most conventional construction materials, and so either as additives or assembled into a bulk material their use in industry is only increasing. If successful, the mass production of CNTs as a by-product of methane cracking adds another value stream to offset the initial investment of the cracking apparatus.
Cost is not the only barrier to the mass adoption of methane cracking. The complex nature of the technology setup, collecting both the hydrogen and the high-quality carbon products, is a significant hurdle in the way of commercialisation and packaging the technology. Levidian is a company that is targeting just that, offering a solution rather than a ‘method’ in the space. Levidian’s LOOP technology is an all-in-one methane-cracking device. At the size of a small shipping container, their compact systems are designed to easily integrate with existing infrastructure.
Levidian’s LOOP uses microwave plasma pyrolysis7. This differs fundamentally from the plasma pyrolysis described above. Instead of using the plasma to heat the methane, the plasma is formed from the methane: the microwaves generated in LOOP directly ionise the methane gas, creating a plasma. The result of this is a more efficient form of pyrolysis methane cracking compared to conventional plasma pyrolysis methods. The relatively low reactor temperature and pressures also lend more control of the methane cracking environment - Levidian has been able to optimise the conditions so that the solid carbon is formed directly into high-quality graphene.
Graphene and its exceptional strength, conductivity, flexibility, and transparency have sparked immense interest across various industries. From the automotive industry to MedTech the potential uses of this material in industry are still relatively untapped. At the moment, high-quality graphene is priced at around £850 per kilogram and with increasing demand Levidian offer both environmental and economic incentives for its methane-cracking LOOP system.
Methane cracking looks to be to a win-win process for both industry and environmental legislators. Being able to break down a potent greenhouse gas, produce green hydrogen and manufacture highly sought-after carbon nanomaterials paints a positive picture when looking towards lofty environmental targets, and as evidenced here, it seems mass commercialisation is on the horizon.
This blog was originally written by Amin Zouine.
References
Thomas is an associate patent attorney with experience in drafting and prosecution of patent applications for a range of European and international clients in the fields of chemistry, biochemistry, pharmaceuticals and materials. He has also worked on FTOs and attended proceedings before the EPO. Thomas holds a Masters (MChem) and doctorate (DPhil) from the University of Oxford in which he specialised in biocatalysis.
Email: thomas.lonsdale@mewburn.com
Our IP specialists work at all stage of the IP life cycle and provide strategic advice about patent, trade mark and registered designs, as well as any IP-related disputes and legal and commercial requirements.
Our peopleWe have an easily-accessible office in central London, as well as a number of regional offices throughout the UK and an office in Munich, Germany. We’d love to hear from you, so please get in touch.
Get in touch