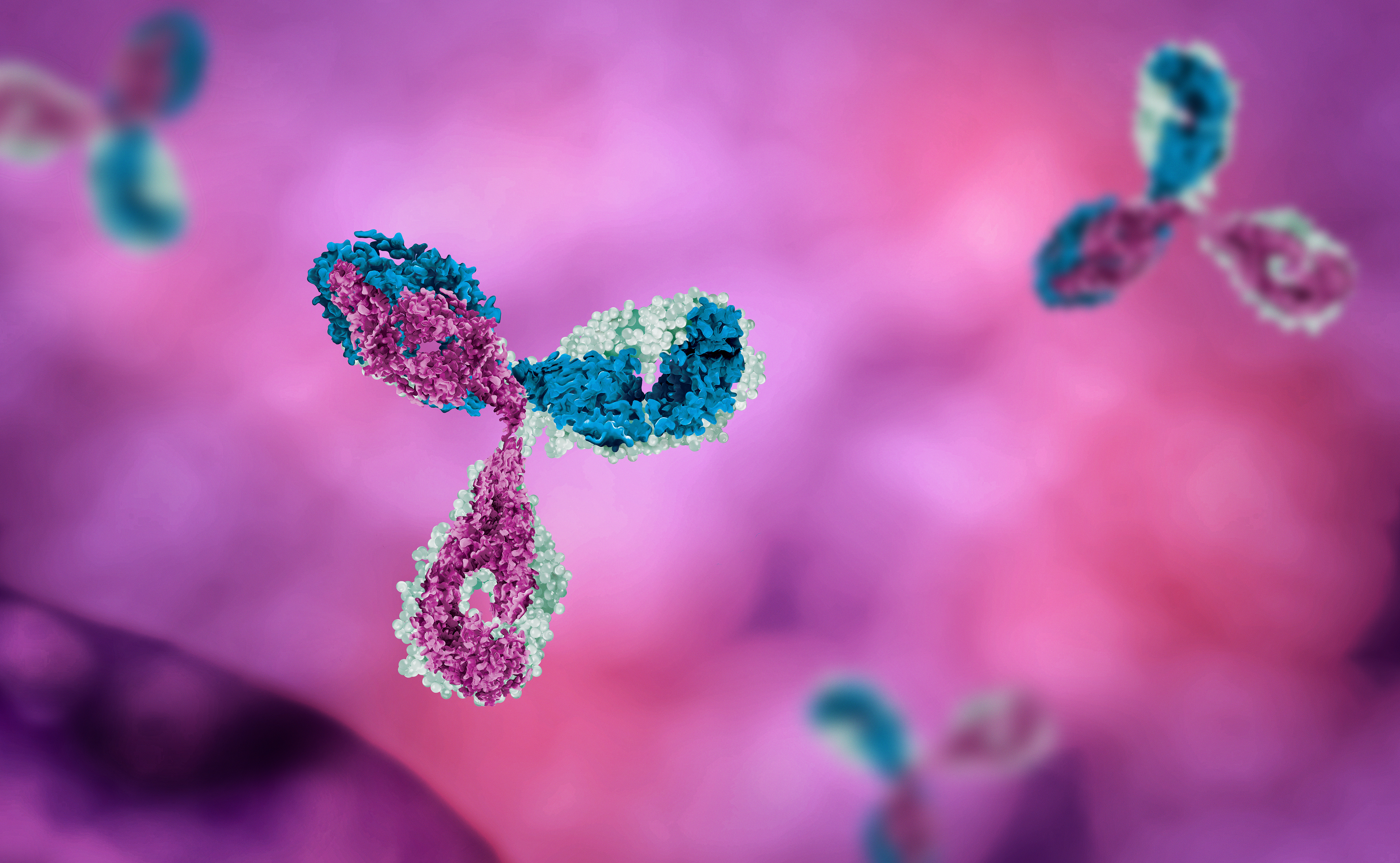
In vivo CAR therapies build on the success of standard CAR treatments and aim to overcome the limitations of standard CAR therapy. Here we consider the drawbacks associated with traditional CAR therapy, and assess how advances in gene therapy are enabling new avenues for CAR therapy.
Chimeric antigen receptors, or “CARs”, have shown impressive results in cancer treatment, particularly haematological malignancies. In their simplest form they contain an antigen binding domain (typically an scFv), a transmembrane domain and at least one intracellular signaling domain. In the commonly used autologous approach, immune cells (traditionally T cells) are collected from a patient and engineered ex vivo (i.e. outside the patient’s body) to express the CAR. The cells are then readministered to the patient, where the antigen binding component of the CAR recognises and binds to specific antigens on cancer cells. This triggers activation of the intracellular signaling domain, causing the CAR-T cells to proliferate and secrete cytokines to kill the cancer cells.
Six CAR therapies have been approved by the FDA since 2017[i]. So far, all of the approved therapies are designed to treat blood cancers and target either CD19 or BCMA on the surface of B cells (a type of white blood cell). The field remains active, with hundreds of ongoing clinical trials investigating other target antigens and engineering cells other than T cells, such as CAR-NK cells.
Despite these successes, CARs face many issues. These include high incidences of systemic toxicity, such as cytokine release syndrome (CRS) and neurologic effects or immune effector cell-associated neurotoxicity syndrome (ICANS).
Other problems are associated with their complex manufacturing process. Collection of cells from patients, ex vivo gene-editing and expansion and infusion back into patients all pose significant risks, as well as challenges to developing standard operating procedures for such a highly personalised process. The complexity means the processes are expensive, resource-intensive, and require substantial technical expertise resulting in the treatment only being available at a limited number of specialised centres worldwide. Another inherent problem is the lag time between removing the cells and re-infusion, which is time that may not be available to the most seriously ill patients.
In an attempt to combat the lag time for these autologous therapies, universal allogeneic approaches which are available immediately (off-the-shelf or ‘OTS’ cells) are being developed. However, allogeneic cell therapy comes with its own limitations due to the risk of risk of graft-versus-host disease (GvHD) and confidence in allogeneic CAR-T cells has been dampened by safety concerns following a death in a clinical trial.
In vivo CARs aim to overcome the challenges associated with conventional CARs by generating CAR-T cells inside the patient, i.e. without the need to remove cells from the patient, engineer them and subsequently infuse them back into the patient. This is achieved by encapsulating CAR-expressing plasmids into nano-delivery systems, which are administered systemically to the patient.
The nano-delivery systems are designed to specifically target the CAR to the correct immune cells, e.g. T cells. Vectors are designed to be selective for a specific immune cell surface receptor, such as CD3, CD4 or CD8. This avoids the CAR being delivered to non-immune cells, including cancer cells, which can have fatal consequences. The incorporation of high-affinity targeting molecules such as scFv domains or designed ankyrin repeat proteins (DARPins) into the nano-delivery systems has greatly improved cell selectivity[ii],[iii],[iv]. Furthermore, the manufacture of the carriers can be standardised which reduces the complexity, time and cost of the manufacturing process.
The CAR can be delivered in vivo using viral vectors or non-viral delivery systems.
Viral vectors
Viral vectors have the benefit of high transfection efficiency, but can also lead to cellular toxicity and reduced immunogenicity, and the amount of genetic material they can deliver is limited.
Standard CAR therapies commonly use lentiviral (LV) vectors, pseudotyped with vesicular stomatitis virus (VSV-G) which allows cell entry via the LDL receptor. As this receptor is found on many cell types, LV vectors have a broad tropism.
AAV vectors are also commonly used to deliver genetic material to target cells in a clinical setting, and have a lower risk of toxicity relative to other viral vectors. Recently, tumour regression was achieved in a mouse model of human T-cell leukaemia with an in vivo CAR using AAV delivery[v]. The viral vector encoded an anti-CD4 CAR composed of a scFv specific for the extracellular domain of the CD4 antigen fused with CD28, 4-1BB, and CD3 zeta cytoplasmic signaling domains. The T cells were shown to be reprogrammed and sufficient CAR T cells were generated in vivo after a single infusion. In vivo CAR generation has also now been demonstrated in mouse models for all vector platforms[iv].
Non-viral delivery
The non-viral routes can be divided into physical and chemical techniques. Physical techniques include electroporation, injection, laser irradiation and gene guns. Chemical techniques include lipid-based nanoparticles (LNPs) – an attractive option due to their approval for use in the clinic (most notably the SARS-CoV-2 mRNA vaccines) – and nanocarriers (NCs). NCs are complexes of negatively charged nucleic acid and positively charged poly(β-amino ester) (PBAE) polymer. Following entry of the NC into the cell via endocytosis, the nucleic acid component then escapes the endosome and enters the cytosol. Cationic polymers have been shown to successfully generate in vivo CAR-T cells with no systemic toxicity[vi],[vii].
Depending on the delivery mode chosen, the CAR will be either transiently or stably integrated into the target T cell. For example, lentiviral vectors and transposase-enabled NCs perform stable integration. This means single injections of the vector can be sufficient to generate persistent CAR-T cells in vivo. In contrast, for transient gene transfer multiple doses will be required to maintain a sufficient level of CAR-T cells.
Future work in this area will further develop both the cargo and the delivery systems to optimise efficacy, safety and cost. The application of CRISPR to generate the CAR in vivo could be an exciting future development. This is a promising approach as CRISPR already has a proven track record in vivo for other applications. As the field develops, in vivo CARs are likely to be applied to diseases beyond cancer, with early-stage research already showing promising results in cardiac injury[xii].
In vivo CAR therapies look set to have a promising future as the go-to immune-cell therapy for cancers and beyond.
[i] Chen YJ et al. CAR-T: What Is Next? Cancers (Basel). 2023 Jan 21;15(3):663
Fay is a patent attorney in our life sciences team. She has an undergraduate BSc degree in Biochemistry from the University of Bristol and a PhD in Biological Sciences from the University of Cambridge. During her undergraduate degree, Fay undertook a one year industry research placement in synthetic biochemistry, developing alternative protein expression systems in bacteria. Her doctoral research focused on mitochondrial dysfunction, particularly mitochondrial metabolism during ischaemia-reperfusion injury and heart transplant. Fay joined Mewburn Ellis LLP in 2019.
Email: fay.allen@mewburn.com
Our IP specialists work at all stage of the IP life cycle and provide strategic advice about patent, trade mark and registered designs, as well as any IP-related disputes and legal and commercial requirements.
Our peopleWe have an easily-accessible office in central London, as well as a number of regional offices throughout the UK and an office in Munich, Germany. We’d love to hear from you, so please get in touch.
Get in touch