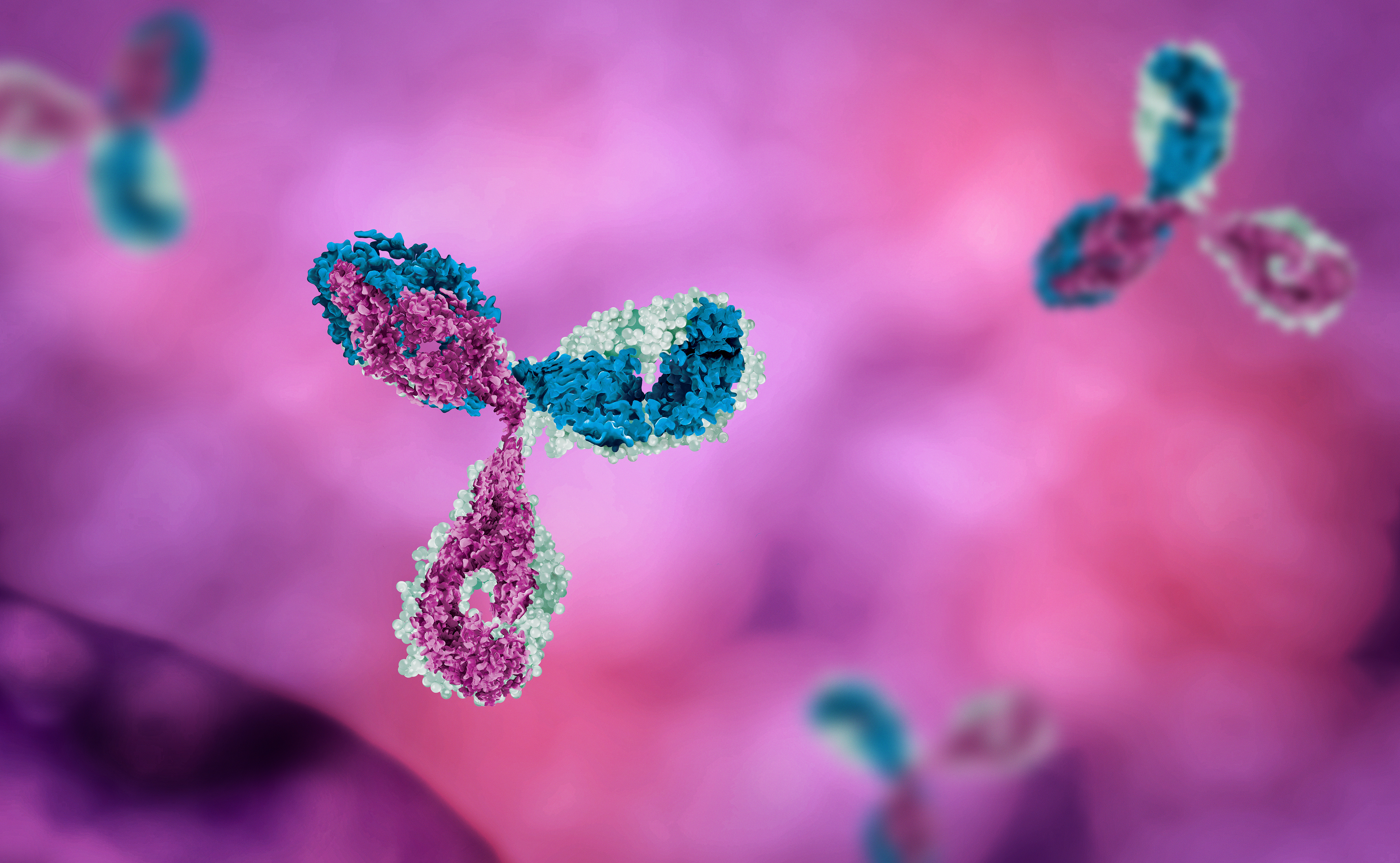
Millions of people around the world depend on implanted medical devices, for all sorts of reasons – however, one type of medical implant that is critical to ensuring many patients’ longevity is the pacemaker.
Pacemakers are typically implanted in the chest or abdomen of a patient and serve the function of an artificial sinus node – the node of the heart that creates a steady pace of electrical impulses to control heartbeat – in patients suffering from abnormal heart rhythms (arrhythmias). Instead of relying of the sinus node, patients with pacemakers are reliant on electrical impulses sent by the pacemaker which tell the heart to contract and produce a heartbeat. These pulses can either be sent at a fixed rate, or on demand, depending on the needs of the patient, and the specifications of the pacemaker.
With delivery of electrical impulses being the pacemaker’s main job, the power source of the pacemaker is clearly critical to its function. Powering pacemakers has been a significant technical issue since their first conception: the earliest pacemaker-like device, developed by New York cardiologist Albert S. Hyman in the early 1930’s, used a portable hand-crank generator connected to a current-interrupting device to deliver electric current to the right auricle of the heart via a long needle. However, it was apparent that manually-generated power wasn’t an effective way forward for long term use, and so, the battery-powered pacemaker was eventually born in the form of the Elmqvist-Senning pacemaker. This pacemaker was developed by a surgeon Ake Senning and a physician inventor Rune Elmqvist, and first implanted in a human on October 8, 1958. It used a nickel cadmium battery, which required frequent transcutaneous recharging, and the first device implanted lasted only 3 hours. Both its poor battery capacity and low durability were a significant impediment to its clinical adoption.
Fortunately, pacemaker technology has moved on significantly since the 1950’s. Nowadays, most modern pacemakers use lithium primary batteries since they meet the requirements of long life, low drain current and voltage characteristics, and the lifespan of these pacemakers is typically in a range of from about 6 to 10 years. However, given the steadily rising average life expectancy across the world (life expectancy in England in 2020 was 78.7 years for males and 82.7 years for females), this means that for many patients requiring a pacemaker, one, two, or three or more invasive pacemaker-change operations may be required in their lifetime after the initial implantation.
One way forward to extend the lifetime of these critical devices is to consider the possibility for self-powering the device by means of in-vivo energy harvesting. However, there are significant complexities to achieving this goal: suitable devices must be biocompatible (to avoid rejection after implantation) and durable (to ensure that the lifetime of the device isn’t limited by other, non-power-related factors). They must also produce a sufficient charge to power these complex medical devices with relatively significant power needs, and this is where the main challenge lies.
The endocardial pacing threshold energy for the human heart is around 0.377 μJ. There have been some developments in technologies meeting this minimum energy harvesting criteria, with triboelectric technologies (based on the triboelectric effect, where certain materials become electrically charged after they are separated from a different material with which they were in contact) leading the way:
However, developments in this field are not limited to triboelectric materials only – piezoelectric materials which generate a charge in response to applied mechanical stress also offer strong potential. For example, Azimi et al reported in 2021 the development of a biocompatible and flexible piezoelectric polymer-based nanogenerator (PNG) comprising composite nanofibers of poly(vinylidene fluoride) (PVDF) and a hybrid nanofiller made of zinc oxide (ZnO) and reduced graphene oxide (rGO). This nanogenerator was found to yield an energy of 0.487 μJ upon implantation in the context of a large animal model (dog).
With multiple technologies now meeting the minimum endocardial pacing energy threshold requirements, it seems it that great strides are being taken towards the goal of self-powered pacemakers. However, we still have some way to go before the advent of human-ready self-powered devices: on top of this minimum energy requirement, the latest generation of pacemakers have much higher power demands, requiring additional energy for functions such as performing analysis of the underlying cardiac rhythm, checking sensitivity and changing pacing thresholds, as well as functions such as transmitting data to remote monitoring systems – e.g. via bluetooth to a phone for app based monitoring. As pacemaker technology constantly evolves, it’s a never ending race for energy harvesting technologies to keep up, and so it may be a while until we meet the goal of ensuring that pacemakers can have a lifespan comparable to that of the patients themselves.
Isobel is a Partner and Patent Attorney at Mewburn Ellis. She is an accomplished UK and European patent attorney whose technical expertise spans a wide range of technical fields in the mechanical engineering and materials engineering spaces. Her work extends across the full IP lifecycle: she has extensive experience in original drafting and patent prosecution work, as well as a keen interest in opposition and other contentious matters.
Email: isobel.stone@mewburn.com
Our IP specialists work at all stage of the IP life cycle and provide strategic advice about patent, trade mark and registered designs, as well as any IP-related disputes and legal and commercial requirements.
Our peopleWe have an easily-accessible office in central London, as well as a number of regional offices throughout the UK and an office in Munich, Germany. We’d love to hear from you, so please get in touch.
Get in touch