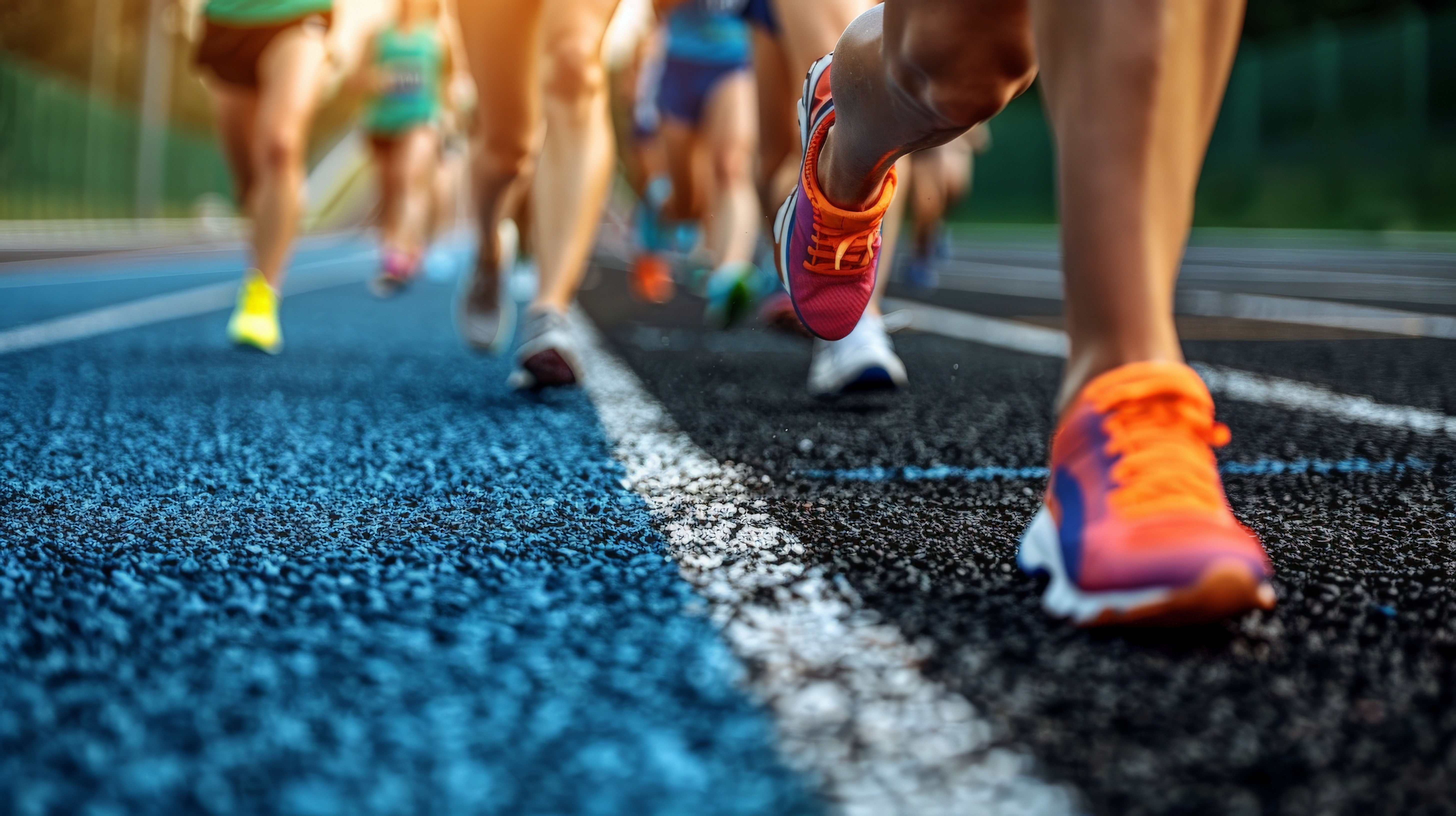
Rising global temperatures and rising populations threaten farmers’ ability to grow the crops we need to sustain ourselves. But, as Forward finds out, scientists are seizing the initiative.
Forward: features are independent pieces written for Mewburn Ellis discussing and celebrating the best of innovation and exploration from the scientific and entrepreneurial worlds.
In the midst of a heat wave in Australia, swathes of wheat stand in a temperature controlled glasshouse, illuminated in a sea of deep pink, each row of plants tended to by a row of LEDs overhead. Here, at the Hickey Lab in Brisbane, researchers are investigating what is in essence a very simple concept: that plants can be flooded with light to keep them from sleeping and to trick them into flowering more quickly.
The concept, dubbed ‘speed breeding’, isn’t new. ‘The idea was first carried out by NASA- funded research back in the 1980s, when they were aiming to grow wheat in outer space,’ says Associate Professor Lee Hickey, a crop geneticist within the Queensland Alliance for Agriculture and Food Innovation at The University of Queensland. ‘Over the years, the technique has been improved and operations streamlined. Now it’s a highly efficient breeding tool. We can achieve up to six generations per year, and we’ve adapted those methodologies to a whole range of species.’
Associate Professor Lee Hickey
So why is there the need for speed in bringing new plant varieties forward? Well, the security of our staple crops is under threat. The ongoing heating in our climate means that the conditions in which we currently grow our food are changing drastically. That – along with a rising population, rapidly evolving plant diseases and vanishing land resources – means that the principle sources of our basic foodstuffs have a lot to contend with in the near future.
As a result, new and improved genetic varieties of crops are needed, including those that are capable of withstanding drought, disease, higher temperatures and more. Typically, that’s achieved when plant breeders take two different varieties of a crop and crossbreed them in an effort to find and pass on desirable traits. However, getting from those initial breeding stages to growing a new crop, testing it in the field and analysing the data can take between 8 and 15 years. ‘Speed breeding is really about trying to shrink that down,’ says Hickey. ‘You can do the field testing faster because you can generate those new materials much faster.’
The simple fact is that recent evolutionary history and more recent interventions mean that the diversity of genes in the crops we grow is lacking. ‘The materials we have in our breeding programmes have gone through a massive genetic bottleneck in the last hundreds of thousands of years – and more recently with modern breeding. This means we need to go back to that genetic diversity and find new genes and traits,’ explains Hickey.
While much of that genetic diversity is preserved in seed banks around the world, challenges remain. ‘The materials are really wild. They’re not like the wheat crops we grow in the field, but they do have a lot of good attributes. So we have to find the materials that have got the good genes and separate those out from all of the bad genes.’
The advent of genomics and genome sequencing is making the job of plant breeders much easier. Genomic prediction, a technology originally developed for breeding animals, allows us to sequence a plant and use algorithms to predict its performance using massive historical data sets. ‘That means, in a way, plant breeding is becoming less reliant on phenotyping – that is, doing the trials in the field. We can use all of our data, make predictions and cross the best plants with the best,’ says Hickey.
Alongside the rise of genomics, the development of another technique known as gene-editing has given researchers the ability to precisely alter an organism’s DNA by the insertion, deletion or replacement of DNA in a specific part of the genome. In recent years, the development of a new method known as CRISPR-Cas9 has allowed gene editing to be carried out simply and inexpensively. With that, gene editing has become much more accessible, bolstering innovation within the field.
"We can use all of our data, make predictions and cross the best plants with the best"
Now that researchers have the power to change the genome, the question is simple: what to change? The answer depends on what result you want. Some plant traits are controlled by individual genes, meaning their modification provides very specific results. This particularly applies to genes that are responsible for susceptibility to certain diseases; turning a certain gene off means that the plant will be naturally more resistant.
But other traits require more complex interventions. Recent research has shown that by tweaking the activity of transcription factors (master regulators that turn other genes on and off) you can improve a plant’s performance. In the past, companies attempted to take these transcription factors and overexpress them. ‘That wasn’t very successful – it was kind of like giving the master regulators steroids so they were on all the time,’ says Professor Cristobal Uauy, a geneticist at the UK’s John Innes Centre who is working on wheat. On the other hand, by increasing the gene’s expression a little bit – say, by 10% – many aspects of a plant’s performance can be improved. Using gene editing, scientists can tweak the promoter sequence – the regulating sequence of the gene that will in turn become the master regulator – to get the same effect.
One innovative agritech company working to identify useful gene targets, as well as investigating the effects of modifying transcription factors, is Yield 10 Bioscience. By using its next-generation platform GRAIN – a predictive tool which aids researchers in finding complementary combinations of genes to be edited (what they call “smart targets”) – the company works on investigating gene targets that when altered will produce crops with higher stress tolerances, higher yields and more. Meanwhile, ‘plant-based technology company’ Calyxt is using its proprietary gene-editing technology to improve the nutritional value of food. This includes, for example, altering the genes of wheat to increase the dietary fibre content three-fold. It is also working on enhancing the levels of saturated fat in soybeans to provide a sustainable alternative to palm oil.
Yet, while gene editing is an immensely powerful tool, there are still some regulatory hurdles that need to be overcome in order to get gene-edited plants into the hands of farmers. The major crop-exporting nations (the US, Australia and Brazil) have already approved its use, but importers have been more cautious. In 2018, an EU court ruled that gene-edited strains cannot be given to farmers because they are considered genetically modified.
Uauy admits that, technically, such strains may indeed be ‘modified in their genetics’. ‘But then every single plant in the world is a GMO,’ he continues. ‘When you grab a spike of wheat, all of the grains are the sons and daughters of that plant. Each one of those grains will have between 90 and 100 mutations. When you look at a field of wheat, it’s full of mutations – hundreds of thousands of them. But to find the mutation you want is super tricky. That’s what gene editing allows you to do.’
"When you look at a field of wheat, it’s full of mutations – hundreds of thousands of them. But to find the mutation you want is super tricky. That’s what gene editing allows you to do"
Uauy also points out that conventional breeding methods introduce far more mutations than gene editing ever could. ‘Breeders today have to do so much work to find the mutation they want, so they use chemicals to create lots of mutations. So you go from 90 mutations – which are present in any seed – to about 500,000. Because we’ve eaten these plants for many years, we consider them to be safe.’
It’s not just about being able to alter crops to ensure they survive the coming challenges. We must also understand the diversity and biology of pathogens to be able to develop effective weapons against them. ‘On a global basis, about 40% of crop yield is lost to pest and diseases, and the burden of loss is highest in developing nations where the awareness of the diseases maybe isn’t as strong,’ says John Jones, head of the Cell and Molecular Sciences department at The James Hutton Institute in Scotland.
New techniques being developed by Jones’ colleagues allow them to sequence DNA on parts of the genome that they’re interested in – the resistance genes – and forget about the rest. ‘These things can be incredibly difficult to work with, they’re very complex organisms with very intimate interactions with their host, but we’ve learned so much about the biology of pathogens by sequencing the genomes.’ That knowledge, Jones explains, can be combined with the expertise in the agrochemical industry to focus the search for new agrochemicals on pathways and proteins that exist only in pathogens, removing some of the off-target effects they might have.
While our plants need to survive, they also need to thrive – and in recent years crop yields have begun to plateau. With 10 billion mouths to feed by 2050, scientists need to look at new ways of increasing food productivity to be able to meet the world’s nutritional demands – and simply clearing new land for agriculture isn’t an option. At Lancaster University, Dr Elizabete Carmo-Silva is exploring one way of doing that: improving photosynthesis. As photosynthesis is the primary determinant of crop yield, improving the sub-processes involved may make it possible to improve crop yields by more than 40%.
‘What I’m fascinated by is Rubisco [Ribulose bisphosphate carboxylase/oxygenase] and its regulation,’ explains Carmo-Silva. ‘This enzyme is basically the starting point of carbon assimilation into the plant. All of the carbon in the biosphere goes through the active site of this particular enzyme.’
In the shade, Rubisco activity decreases. When the leaf transitions from the shade into sunlight, Rubisco’s activity levels begin to increase, making the process of photosynthesis faster too. But that jump from low activity to higher activity takes time. ‘That transition might take more than ten minutes. Our goal is to make this process a lot faster,’ says Carmo-Silva.
To do that, her team is looking at an enzyme that activates Rubisco, called Rubisco Activase. Differing forms of that enzyme are present in different varieties of plants, and some of them are better at activating Rubisco than others. ‘When we identify versions of the enzyme that are faster, we can then tell breeders to make a cross between one plant that has the faster enzyme and an elite breeding cultivar.’ The team is also looking at using gene editing to make changes to the building blocks of the activase enzymes. ‘It’s still early days, but we have found one amino acid that we can switch, which makes the enzyme operate much better,’ says Carmo-Silva.
What these researchers share is not only a concern for the wellbeing of our food supply, but also a frustration that the available technology isn’t being exploited to its full potential – for instance due to lingering concerns about genetically modified food. ‘I have hopes that things will change and that people will become more open to different technologies. Because at this stage, we are going to run out of food, and our plants are not going to be able to cope with global warming,’ says Carmo-Silva.
After all, using these tools would make our agriculture more green, not less, says Cristobal Uauy. ‘This could allow you to have more organic agriculture. That’s what scientists want to do – we want less pesticide, lower inputs. And for that, we need these tools.’
Simon Kremer, Partner and Patent Attorney at Mewburn Ellis comments:
"The development of agriculture from its first beginnings has always gone hand in hand with technical innovation. It is enormously exciting to see the many different ways that the latest technologies, genomic insights and computations tools are being brought to bear on the ever more pressing problem of declining crop yields."
Written by Patrick Hughes
Images provided by Hickey Lab
Simon has more than 25 years’ experience working as a European and UK patent attorney working in the life sciences sector. He provides clear and practical advice on all aspects of patents and other IP rights including drafting, prosecution, inter partes proceedings and due diligence. He is regularly involved in oppositions and appeals at the European Patent Office and covers a wide variety of technical fields, including physical biochemistry, therapeutics, diagnostics, genetics, chemistry and mechanical subject matter.
Email: simon.kremer@mewburn.com
Our IP specialists work at all stage of the IP life cycle and provide strategic advice about patent, trade mark and registered designs, as well as any IP-related disputes and legal and commercial requirements.
Our peopleWe have an easily-accessible office in central London, as well as a number of regional offices throughout the UK and an office in Munich, Germany. We’d love to hear from you, so please get in touch.
Get in touch