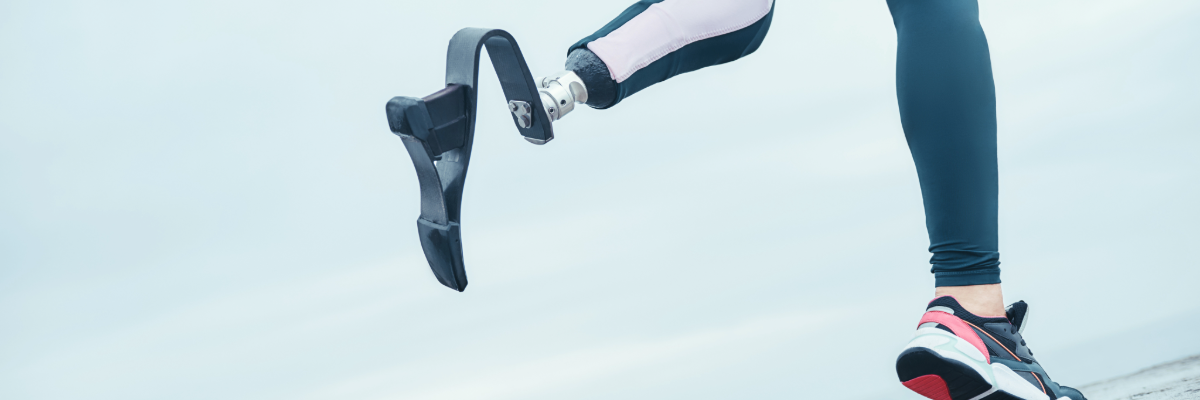
A fusion energy pilot plant will be up and running in ten years, says Tokamak Energy chairman Chris Martin. A commercial 500MW reactor will follow shortly. We are on the brink of the fusion age.
Written for Mewburn Ellis by Charles Orton Jones.
The old line about fusion is that it’s always 30 years away. Is this still true?
The man who knows is Chris Martin, chairman of Tokamak Energy. The company, a spin-off from UK Atomic Energy Authority, is focused on delivering clean, limitless fusion energy – the power of the sun and stars. It is a world leader in two transformative technologies: the compact spherical tokamak and high temperature superconducting (HTS) magnets, which are essential to confine the plasma of fusion fuels. These technologies have been selected by the UK government for its flagship Spherical Tokamak for Energy Production (STEP) programme and are considered the most efficient and cost-effective route to commercial fusion energy.
So how close are we to fusion?
Martin delivers this bold update: ‘Our mission is to deliver fusion energy in the 2030s. We think 2034 for a demonstrator is realistic. And someone will have a commercial reactor ready in the second half of that decade.’
Are there any obstacles clearly preventing this?
Martin’s answer is full – but the short version is ‘no’. He says: ‘There is work to do improving the lithium blankets, which absorb the neutrons. That is an engineering challenge.’ Adding: ‘The other area is plasma-facing materials. These are the bits that aren’t covered by the lithium, but nonetheless are going to get high-energy radiation. We need to develop things like vanadium steels able to last 50 years in that environment. But people who specialise in steels are working on it.’
Can we therefore declare the road to fusion is clear? ‘That is an accurate way of describing it, yes,’ says Martin.
Chris Martin, Tokamak Energy Chairman
This is profound news. Fusion promises limitless, cost-effective and, above all, clean energy. One kilogram of fusion fuel releases the same amount of energy as burning around 10 million kilograms of coal, with no harmful emissions.
And it’s safe. The fundamentals of fusion make it impossible for a Chernobyl or Three Mile Island incident to occur. Unlike fission, which splits heavy atoms in a chain reaction that could run away from itself without proper safety controls, fusion combines the lightest of atoms in a way that fizzles out as soon as you turn off the power.
‘The thing about fusion is you have to go to an enormous length to overcome the repellent forces between two hydrogen nuclei,’ explains Martin. ‘You have to heat the plasma to over 100 million degrees Celsius. You need a certain pressure. You have to hold them together for a reasonable amount of time and the moment you relax those parameters, it stops. So it is the opposite of fission.’
The waste is partly reusable: ‘The neutronics of the lithium, absorbing the neutrons, produces tritium, which is one of the fuels a fusion plant needs, so we breed our own fuel,’ says Martin.
Plant construction is simpler in many ways than fission. ‘Much of what you do with fission is over-engineering to avoid meltdowns and to protect it. You need to deal with radiation. The early fission reactors built after the war were cheap because they didn’t have that stuff.’
So why isn’t the world a little more excited? Bringing the same energy that powers stars to planet Earth is the stuff of science fiction. The answer lies in the chequered history of fusion, which is why Martin’s announcement may get a little lost.
Fusion energy dates back to 1915, when American chemist William Harkins proposed a theory of how hydrogen atoms could combine to create a helium atom. In the 1920s, English physicist Arthur Eddington speculated how stars generate their energy in his work ‘The Internal Constitution of the Stars”. In 1939, Hans Bethe described the processes at work in the fusion reactions that take place in stars, winning him the 1967 Nobel Prize.
There followed an ignoble tradition of overhyping fusion tech, undermining public confidence.
In 1989, Martin Fleischmann and Stanley Pons claimed to have achieved cold fusion – fake news.
In 2002, a team of scientists claimed sound waves in liquid could produce hot bubbles that imploded and caused fusion. After the experiment could not be reproduced, Purdue University launched an investigation into the lead scientist and judged him guilty of ‘research misconduct’ and ‘falsification of the research record’. He was also barred from receiving federal research funding for just over two years.
Charles Seife, in his history of fusion Sun in a Bottle: The Strange History of Fusion and the Science of Wishful Thinking, chronicled the sequence of false dawns. ‘There’s something about fusion that is a little different,’ Seife wrote, ‘that makes generation after generation of scientists deceive themselves.’
Martin’s message is clear: this time is different. The technology is rigorously tested and the science peer reviewed. An international coalition of independent teams is working together to ensure progress is rock solid. His forecasts are based on the evidence.
The main challenge of fusion is in the nature of the reaction. A fusion reactor is many times hotter than the sun to compensate for the lack of gravitational pressure. Hydrogen isotopes deuterium and tritium are heated to more than 100 million degrees Celsius to create a plasma. At these temperatures, in this plasma, the nuclei of the isotopes can overcome their electrostatic repulsion, allowing them to collide and fuse.
Controlling the 100-million-degrees-Celsius reaction is difficult, to say the least. Concrete melts at 1,200°C, steel a 100°C higher. Both are fine for fission reactors, which run at 600°C to 1,000°C. For fusion, something else is needed.
In the 1950s, scientists in the Soviet Union conceptualised a magnetic confinement device, named ‘Tokamak’ after the Russian phrase ‘Toroidal Chamber with Magnetic Coils’. The original tokamak design includes a series of coil-shaped magnets to keep the plasma way from the walls of the reactor. The fields generated by the magnets control the plasma into a doughnut shape, preventing the fuel from escaping and from coming into contact (and burning through) the reactor walls.
‘The idea of creating a magnetic bottle in which you can hold very hot plasma has been around for a long, long time,’ says Martin. ‘The challenge was to have it so that you didn’t use more power in the magnets than you put in. Also to control the plasma in the bottle. The invention of high temperature superconducting magnets has transformed the ability to do that.’
This is the focus of Tokamak Energy. ‘We have incredibly strong magnets, 20 tesla at the face of the magnet, big enough to encompass a fusion reaction. And model-based computer control allows us to modify the current to hold the plasma in position.’
For reference, a magnetic field of 1 tesla is roughly 20,000 to 40,000 times stronger than the Earth’s magnetic field. An MRI machine operates at 1 to 3 tesla.
Tokamak Energy is a champion of spherical tokamaks. Traditional tokamaks are toroidal, or doughnut shaped. The spherical tokamak retains the basic shape with a hole in the middle, but is, as the name suggests, rounder. A common analogy of the plasma flowing around the tokamak is an apple with the core missing. The spherical design places the magnets closer to the plasma, reducing the amount of energy needed – and therefore the cost. Stability is enhanced.
‘The key enablers were high temperature superconducting magnet technology and model-based high-frequency temperature control,’ says Martin. ‘Those things have only come about in the past decade. HTS magnets and making magnets able to lose their superconductivity are things that have only been mastered in the past five years.’
A high-powered laser diagnostic system gives insights into plasma behaviour, measuring temperature and density. An AI-powered digital twin runs pulse simulations to virtually analyse performance. ‘It is the power of AI that will break barriers and accelerate the path to commercial fusion in time to make a difference,’ says Tokamak Energy co-founder David Kingham. ‘It has the potential to knock years off timelines and cut costs, and this will only gather momentum with better technology, training and computing power. This is just the beginning.’
Tokamak Energy passed the 100-million-degrees-Celsius threshold in 2022 – the highest temperature ever achieved by a private-sector company in a spherical tokamak. The benchmark was verified by an independent board of international experts.
The reputation of Chris Martin makes his statements all the more reliable. He’s a titan of the nuclear industry, with a phenomenal track record in other sectors. ‘I started my career in nuclear fission,’ he recalls. ‘I am a chemical engineer by training. I did my PhD at Harwell, when it was part of the Atomic Energy Authority, on nuclear fission reactor safety.’ At the time, the Joint European Torus (JET) project at Culham, nearby in Oxfordshire, was under construction, making the site the one of the best places in the world to study nuclear energy.
He then worked in the oil industry, sold a company in that area, and because of a ‘very broad non-compete’ went to work in biotech. He was the co-founder and CEO of Spirogen, leading to a sale to AstraZeneca. He co-founded ADC Therapeutics (a Mewburn Ellis client, profiled here), launching the successful drug ZYNLONTA for blood cancer, and taking the company to an IPO on the New York Stock Exchange.
He was a board member of a VC fund called Rainbow Seed Fund. ‘Tokamak Energy came and pitched to us and I was the one board member with a specifically nuclear background. They asked me to join, so I’ve been here for pretty much a decade.’
As chairman, his focus is to get the funding Tokamak Energy needs to get from R&D to demonstrator reactor, and then to build a commercial plant.
‘With the right investment, the mid second half of the 2030s for delivery of a commercial demonstration is absolutely feasible,’ says Martin. The technical hurdles are surmountable. There is the small matter of designing a better lithium blanket to absorb the neutrons produced by the reaction: ‘It’s a liquid metal and solid metal and heat transfer challenge. There’s nothing there that we don’t do in other places. Nonetheless, it needs applying in the fusion reactor.’
Some components need upgrading. ‘There are materials sciences challenges, such as producing the vanadium steels. But, again, the large engineering companies such as ATI, which specialises in specialist steels, are engaged and working on those problems. Five years ago, they weren’t, so that’s moved forward.’ Overall, the map to a working, safe, reliable reactor is plotted out: ‘The challenges have been foreseen,’ says Martin. ‘Now it’s a question of doing it.’
The capital outlay will be significant. ‘The kind of number we are talking about is £20bn over a 20-year period. That will be a mixture of private-sector investment, and government funding from the UK and other countries. If we had a billion now we would be able to immediately proceed with building the next high temperature superconductor spherical tokamak.’
This is about the same price as 40 miles of High Speed Rail 2.
As the industry hits milestones, the financial equation becomes easier. ‘Once you get close enough to a working reactor, the government does not need to be convinced, because the private sector will be convinced. But we need state support now, because the second half of the 2030s is still a long way off.’
For now, Tokamak Energy is keeping the accountants happy by selling magnet technology to other sectors, such as renewables and transport. ‘We need revenues and positive cash flows before we get to the fusion prize,’ admits Martin. ‘Potentially, we could do an IPO or do transactions that give investors liquidity. We are very conscious we can’t ask investors to tie up their investment indefinitely.’
In September 2024, Tokamak Energy launched TE Magnetics, a business division to focus on the deployment of high temperature superconducting (HTS) magnets. The products are backed by more than 200 patents, and have applications across fusion, magnetic levitation, propulsion and wind turbines.
Securing intellectual property is vital for Tokamak Energy. Patents are a complex subject for a company with no known date of revenue generation. ‘We patent extensively,’ says Martin. ‘In particular in areas where we are selling products before we reach commercial fusion.’ The company currently boasts a portfolio of more than 200 granted patents. ‘We are also keen to make sure we don’t fall foul of anyone else’s intellectual property. But longer term, we rely on trade secrets,’ he says. The 20-year patent duration is a severe limitation, owing to the long timelines associated with fusion. Some will expire before commercial viability. ‘Ultimately, we have to create a strong intellectual property position that doesn’t rely entirely upon patents.’
The goal is to build mid-sized fusion reactors. ‘It is harder to produce a small fusion reactor, because with things like shielding you need a certain thickness. In many ways, a bigger reactor is easier, because it’s just easier to fit everything in.’ Around 500MW is the sweet spot, says Martin. ‘It’s not very useful to have one big source of energy, as people need energy over a geographic spread. Multiple 500MW reactors allow you to balance the grid and not have too many transmission losses. You get the economies of scale at that size, so it makes sense.’
Tokamak Energy is just one cog in a large fusion industry. But it is one of the most important. Not only does it supply the core technologies, it acts as a hub for the big industry research organisations. It has a memorandum of understanding with UKAEA and General Atomics, which manufactures HTS magnets. It has a deal with Los Alamos National Laboratory, home of the atom bomb, to produce advanced metal-hydride neutron shielding materials. Other partners include Oak Ridge National Laboratory, Sumitomo Corporation, the University of Tokyo in Japan and Sandia National Laboratories – an R&D operation owned by the US Department of Energy.
Even rivals, such as the Korea Superconducting Tokamak Advanced Research (KSTAR) and the French ITER project, aren’t really rivals: ‘I look at the competition and I think our big competitor is global warming. If we are too slow and don’t produce large amounts of economically attractive base load, then people will continue to burn fossil fuels. When I developed cancer drugs, people used to ask which cancer drugs are the competition. I’d say: “Until we’ve cured cancer, cancer is our competition.”’
Fusion is now close enough to play a role in energy planning. Martin puts the energy into context: ‘Solar, wind and hydro will all play a large role in the energy mix of the future. The amounts of these varies enormously by geography around the world. If you model out the energy infrastructure, moving to an energy economy which is 80% electric, then somewhere between 20% and 40% will be fusion powered in the long run.’
A modest forecast, maybe. ‘Wind is very cheap!’ says Martin. ‘And in the desert solar is cheap. So it makes sense to use the best energy source for where you are. I live in Switzerland, where all of our electricity is hydro. It makes sense. The energy mix of the future will be a distributed network of different types, including fusion.’
The promise of fusion energy is tantalising. It changes the path to net zero. It means countries without access to uranium and plutonium can, with the right budget and know-how, become a new type of nuclear nation. Reprocessing waste is revolutionised from the fission era. And no risk of meltdowns is good for our collective stress.
Tokamak Energy is a pioneer creating the energy the world needs for the next era of mankind’s existence.
Fusion has been a false dawn for decades. Now this elusive, infuriating, inspiring technology is on the brink of changing the energy landscape forever.
Alex Savin, Associate and Patent Attorney at Mewburn Ellis, comments:
"Tokamak Energy's bold approach towards IP, particularly its prolific patent filings across a broad range of applications, coupled with their ironclad grip on their trade secrets is a brilliant example to the rest of the nuclear sector of how different types of IP can be leveraged at different times and in different ways to safeguard and improve your commercial position. The launch of TE Magnetics is just one example of Tokamak Energy's creative approach to exploit innovation to provide solutions to today's market so that they can raise revenue and keep pushing ahead to achieve the future goal of limitless clean energy.”
Alex has experience working across all stages of the patent life cycle from invention capture and drafting, through prosecution, to opposition proceedings at the EPO. He is particularly experienced in the fields of optics & photonics and computer-implemented inventions. He also carries out analysis of IP landscapes for freedom to operate (FTO) and IP audit projects, and has experience of helping start-up companies and SMEs build and manage their patent portfolios inventions.
Email: alexander.savin@mewburn.com
Our IP specialists work at all stage of the IP life cycle and provide strategic advice about patent, trade mark and registered designs, as well as any IP-related disputes and legal and commercial requirements.
Our peopleWe have an easily-accessible office in central London, as well as a number of regional offices throughout the UK and an office in Munich, Germany. We’d love to hear from you, so please get in touch.
Get in touch