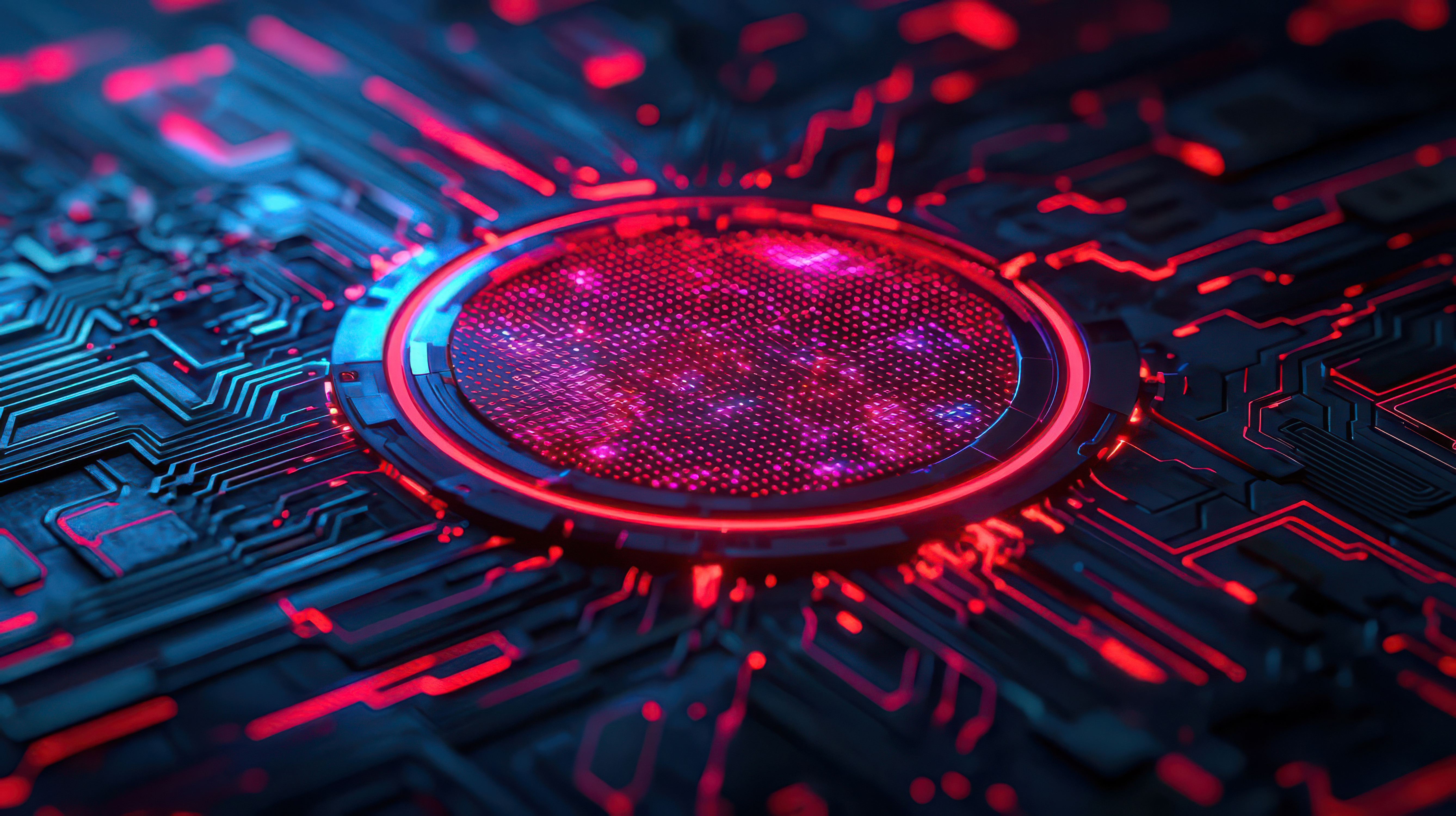
By 2025, the team from secretive quantum computing firm PsiQuantum will have a commercial quantum computer, according to the Financial Times. Having remained mostly silent about development so far, there's an indication that they may be ready to emerge from stealth.
To understand the significance of this announcement, we must first understand the heart of the problem faced by engineers in designing a viable commercial quantum computer. This is how to protect the very fragile quantum ‘bits’, known as ‘qubits’, that are at the core of any quantum computer, where the ‘quantum-ness’ lives (see more in our Forward article Quantum computing: Why qubits have consequences for IP).
Today’s digital computers use processors that ‘speak’ in binary terms. Their language consists of strings of 1s and 0s. They contain billions of tiny transistor circuits manufactured from silicon semiconductor material. They are carefully made to be highly controllable and stable, and each will output a digit 1 or digit 0 faithfully on demand. Not so for qubits, the quantum replacement for the transistor. The quantum-ness of qubits isn’t manufactured by human design, it is ‘made by mother nature’, so to speak, and it follows its own rules. All a human engineer can do is ty to find clever ways to tap into that pre-existing natural, quantum-ness and, critically, to code ‘quantum information’ into it.
Nature’s fundamental particles are super-charged with this desired, built-in this quantum-ness, and quantum computer engineers have largely settled on one of the following two types of particle for use as their qubits: (1) particles of matter; or (2) particles of light. The matter particles come in three flavours which are: individual atoms, individual ions (charged atoms), and electrons. Particles of light simply exist as photons.
Many companies have decided to try matter particles as their qubits. These can be positioned and controlled in various useful ways to physically build-up and operate a quantum processor core. Unfortunately, this susceptibility to control comes at a cost – susceptibility to noise: electrons, atoms or ions are very susceptible to being randomly bumped and jostled by other particles the world around them (see more in our blog Noisy quantum computing: overcoming quantum decoherence). Their delicate quantum information is prone to collapsing into a randomness that’s useless for computing with. The task of the engineer is to design a quantum processor with enough qubits that retain their quantum information for long enough to allow quantum computing operations to be completed using them.
PsiQuantum have adopted the ‘photon qubit’ approach, as opposed to ‘matter qubit’. By being massless, photons don’t interact with heat (or other photons) in their local environment in the way that ‘matter’ particles do. Photons are far, far more likely to retain their quantum information. That said, it’s not a popular approach to quantum computing. Prof. Terry Rudolph, co-founder of PsiQuantum and professor of quantum physics at Imperial College London has described their fundamental approach (of using photons as qubits) in refreshingly unflattering terms:
“…Photonics is the ‘ugly duckling’ of approaches to quantum computing. There are two overarching reasons to try and nurture it into a swan….” (source)
Prof. Terry Rudolph is the grandson of Erwin Schrödinger (the father of quantum mechanics and the originator of the “Schrödinger’s Cat” thought experiment), and the “R” in the well-known (amongst quantum physicists at least!) ‘PBR theorem’ due to Matthew Pusey, Jonathan Barrett, and Terry Rudolph. This is a highly significant theorem on how to interpret deeply counter-intuitive ‘weirdness’ of quantum physics, and it follows in the footsteps of similar philosophical wrangling that his grandfather had with Albert Einstein who thought quantum theory was ‘wrong’ at some fundamental level (Einstein: “…the more success quantum mechanics has, the sillier it looks…”).
Careful thinking also shows through in PsiQuantum’s approach to quantum computing both in terms of its technical implementation and its commercial strategy/realism.
Form the technical perspective, PsiQuantum has not followed the conventional approach to photonic quantum computing. Conventional thought might say that generating many photonic qubits is considered the “easy” part of the problem, and the photonic processor logic circuitry does the “hard” task of performing the quantum computation. An alternative approach, being used by PsiQuantum, is known as “cluster state” quantum computing. Here, a “hard-to-make”, highly “entangled” multi-photon state is sent into an “easy-to-implement” photonic processing circuit. Effective “entanglement” of qubits is a deeply quantum effect in which multiple separated qubits become coordinated in their actions. It is critical to efficient quantum computing and this “cluster state” scheme is viewed as offering a realistic path to commercially scalable photonic quantum computers.
From the commercial perspective, Jeremy O’Brien, Chief Executive of PsiQuantum, has publicly expressed his view that photonics is the only way to deliver a quantum processor with 1 million qubits. That’s thought to be the number needed to provide a general-purpose universal quantum computer. The vast majority of these 1 million qubits would be tasked with protecting the fragile quantum calculations being performed. One million actual qubits, in these circumstances, is ample for a quantum computer to vastly out-gun a conventional computer with several billion transistors. All flavours of qubit, whether matter-particles or photons, face this challenge of how to place so many qubits, and all their connected control circuitry, close enough together to work without producing unwanted noise that will destroy the desired quantum-ness of the qubits. PsiQuantum’s approach appears to be that silicon-based photonic circuits can provide this connectivity. Silicon wafer technology already used in chip manufacture today is well suited to this. A silicon wafer of today can easily contain 1 billion transistors which could in principle be replaced by a photonic circuits for holding 1 million qubits. To some extent then, the problem of scaling-up the technology to many photon qubits is far less of a commercial ‘stretch’ given existing expertise and infrastructure in silicon wafer fabrication and foundries.
That said, many quantum computers look great on paper. Many will be keen to see what technology PsiQuantum has developed.
Andrew is a Senior Associate and Patent Attorney at Mewburn Ellis. He works primarily in the fields of telecoms, electronics and engineering, and specialises in quantum technologies, photonics and ion optics. Andrew has extensive experience of drafting and prosecution, global portfolio management and invention capture to secure a commercially valuable IP portfolio. He also conducts freedom to operate analyses and performs due diligence.
Email: andrew.fearnside@mewburn.com
Our IP specialists work at all stage of the IP life cycle and provide strategic advice about patent, trade mark and registered designs, as well as any IP-related disputes and legal and commercial requirements.
Our peopleWe have an easily-accessible office in central London, as well as a number of regional offices throughout the UK and an office in Munich, Germany. We’d love to hear from you, so please get in touch.
Get in touch